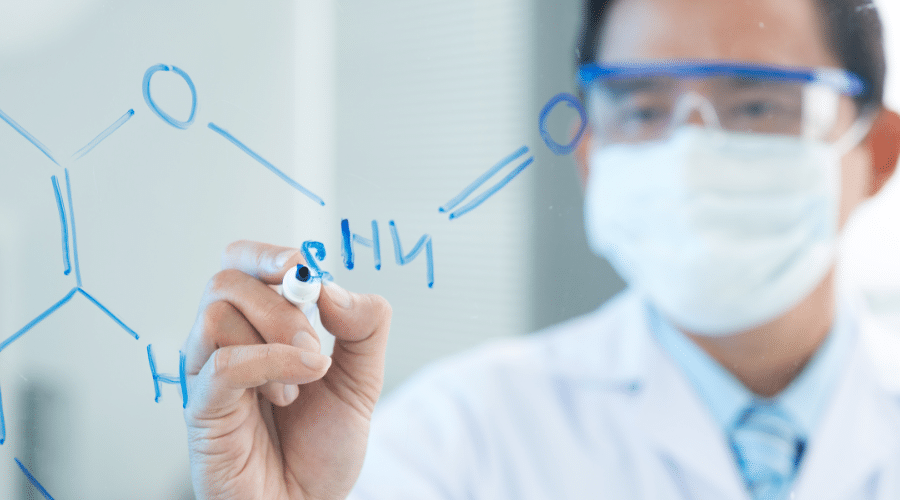
View the full Article on:
Addimmune
Author: Addimmune Staff
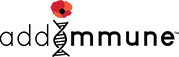
HIV is a breakable mechanism.
Human immunodeficiency virus (HIV) is more fragile than you think. In fact, scientists have found multiple pharmaceuticals which can break the viral life cycle. HIV causes a lifelong infection that inevitably leads to AIDS, a potentially lethal condition, so the diversity of anti-HIV medications available provide a life-saving safety net for millions of infected individuals. However, these drugs must be taken regularly, can have side effects, and – worse – it is possible for HIV to develop resistance to these medications. By understanding how these treatments work, we can see how the science of breaking HIV has progressed over the years, and see what the next few years may hold in store.
At Addimmune, we’re pushing for an HIV cure, and after reading this article, you’ll see how the science of HIV has evolved from the first reported cases of AIDS to our modern understanding of the virus. The first HIV drugs turned HIV from a death sentence to a life sentence, and treatment has improved drastically over the years, leading us to this point and begging the question: how can we translate the effectiveness of HIV drugs into a cure for this viral infection? We believe strongly that HIV can be cured using gene therapy, and the current treatments for HIV form a basis for that argument.
AZT, the first HIV treatment
In the early 80’s, the first cases of HIV and AIDS-related illnesses made headlines, and soon claimed tens of thousands of lives in the US alone. Something had to be done, and fast. By looking through the medicines that had been tested in humans, researchers discovered that Azidothymidine (AZT), a failed cancer drug from the 1960s, was able to inhibit HIV replication. The medication was fast-tracked through the FDA in less than 4 months, and was approved in March 1987, cutting years of regulatory red tape and saving countless lives.
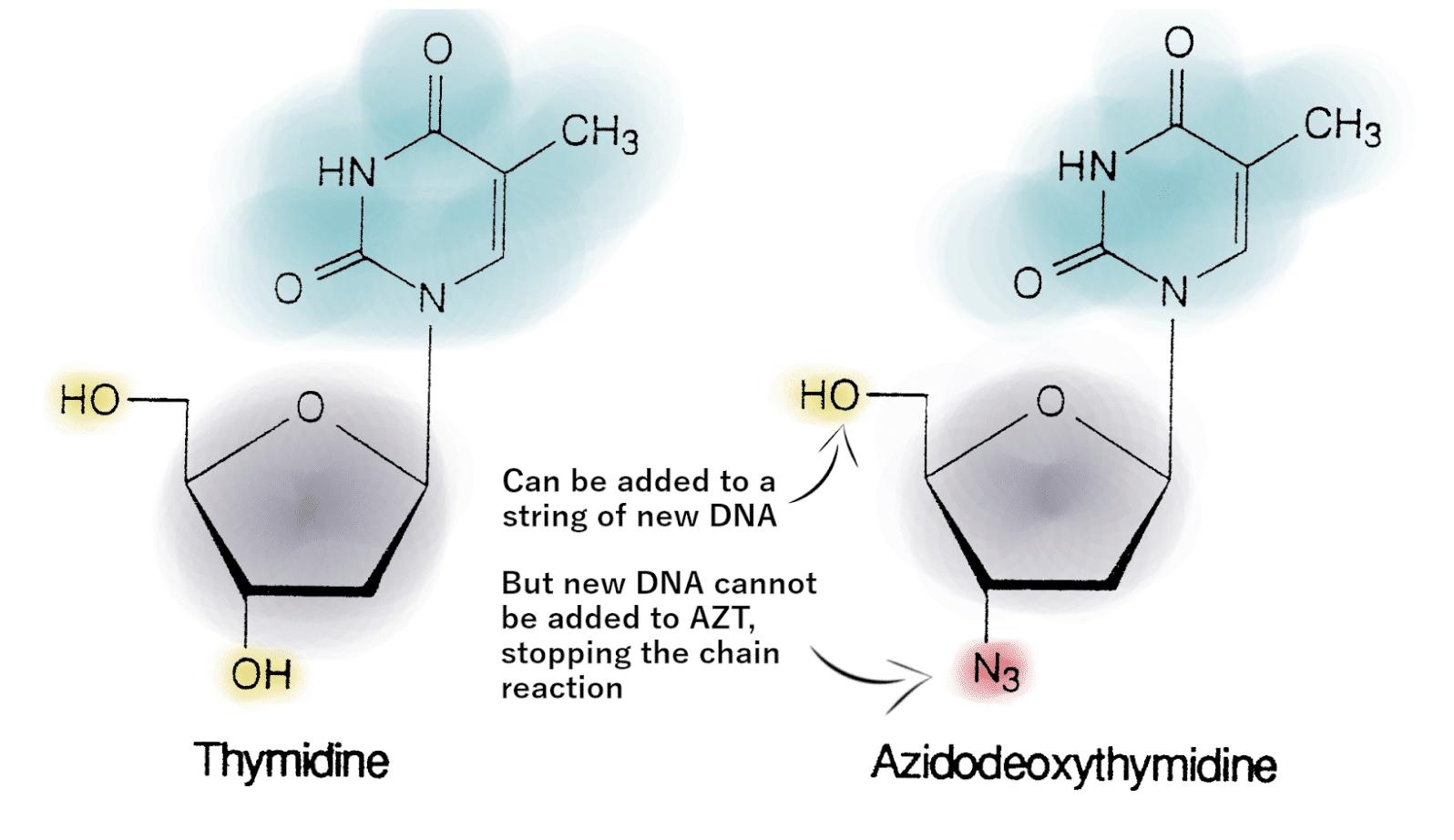
Figure 1: Thymidine, pictured on the left, is a normal part of DNA. AZT, pictured on the right, is very similar, but has an N3 in place of the 3’OH, blocking the ability to add more bases to a sequence of DNA. Photo adapted from Thomas and Segal’s work.
AZT is a chain terminator, meaning that it works by stopping the chain reaction of DNA synthesis. The 5′ OH and 3′ OH serve as points of connection linking the building blocks of DNA, so when AZT is added to a chain of DNA, its N3 group doesn’t allow any more building blocks to connect, stopping DNA synthesis.
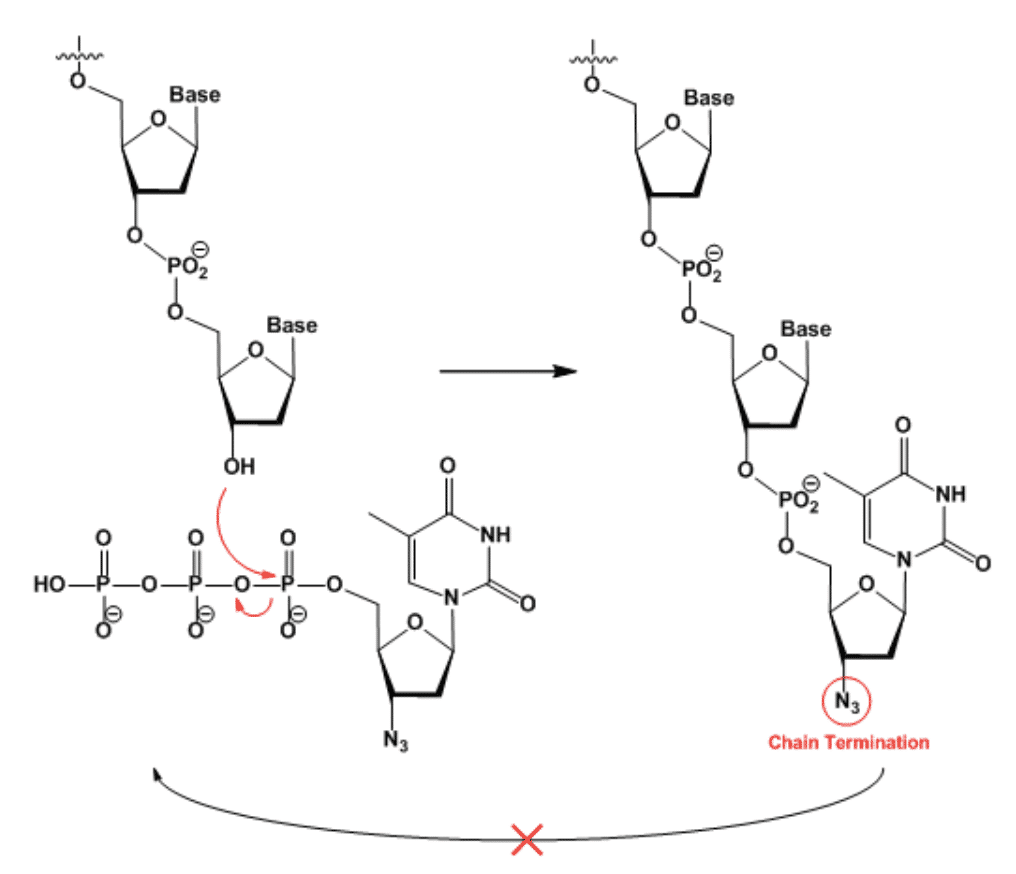
Figure 2: The O-PO2-O bridge between nucleotides is a repeating unit in DNA. When a molecule of AZT is added instead of normal Thymidine, it terminates the DNA polymerization chain reaction. Photo credit to science snail!
The subtle difference between Thymidine and AZT makes it easy to add to a new strand of DNA by mistake, and AZT has a 100-fold affinity for the HIV replication machinery relative to the human replication machinery. Despite its selectivity, AZT had serious side effects such as unusual tiredness or weakness, gastrointestinal pain, swelling, ulceration, fever and more. Despite its side effects, AZT was the only treatment option for a time, and since unchecked HIV infection is inevitably lethal, there is no denying that it saved many lives.
AZT became the first member of the Nucleoside Reverse Transcriptase Inhibitors (NRTI) for HIV, and over the years, 7 other medications have joined the NRTI class. Each new drug offered a better balance between effectiveness and side effects, giving life back to patients who suffered under the debilitating side effects of early medication:
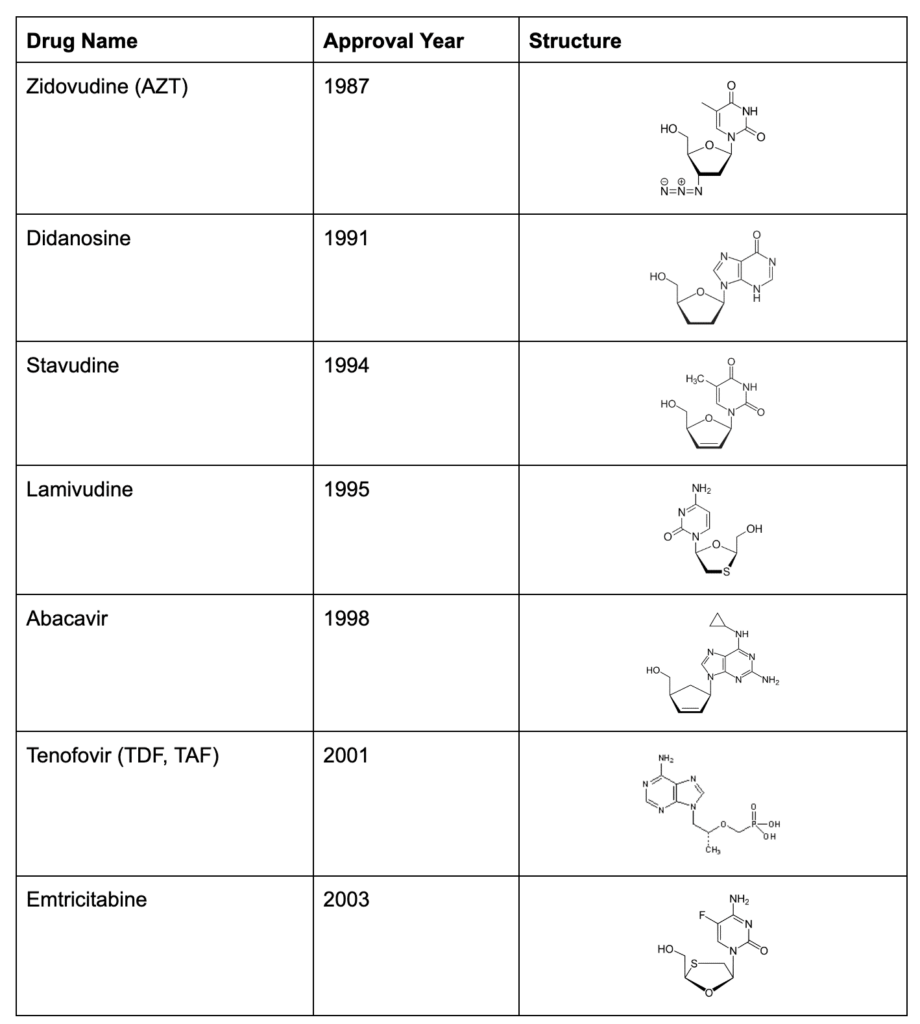
Block HIV in multiple ways: the protease inhibitors
HIV is a small virus with only 9 distinct genes. Compare that to a human with ~20,000 genes, Herpes Simplex 1 virus with ~75 genes, or SARS-CoV-2 with 13-15 genes. HIV uses genetic strategies such as overlapping genes, and genes that encode multiple products, to help compress all of the necessary information into its tiny genome. For example, the gal and pol genes create a fused gal-pol protein that needs to be cleaved by HIV’s protease in order to create the enzymes necessary for the HIV life cycle. In 1995, Saquinavir burst onto the scene as a new class of anti-HIV medication, which could interfere with the HIV protease, pictured below:
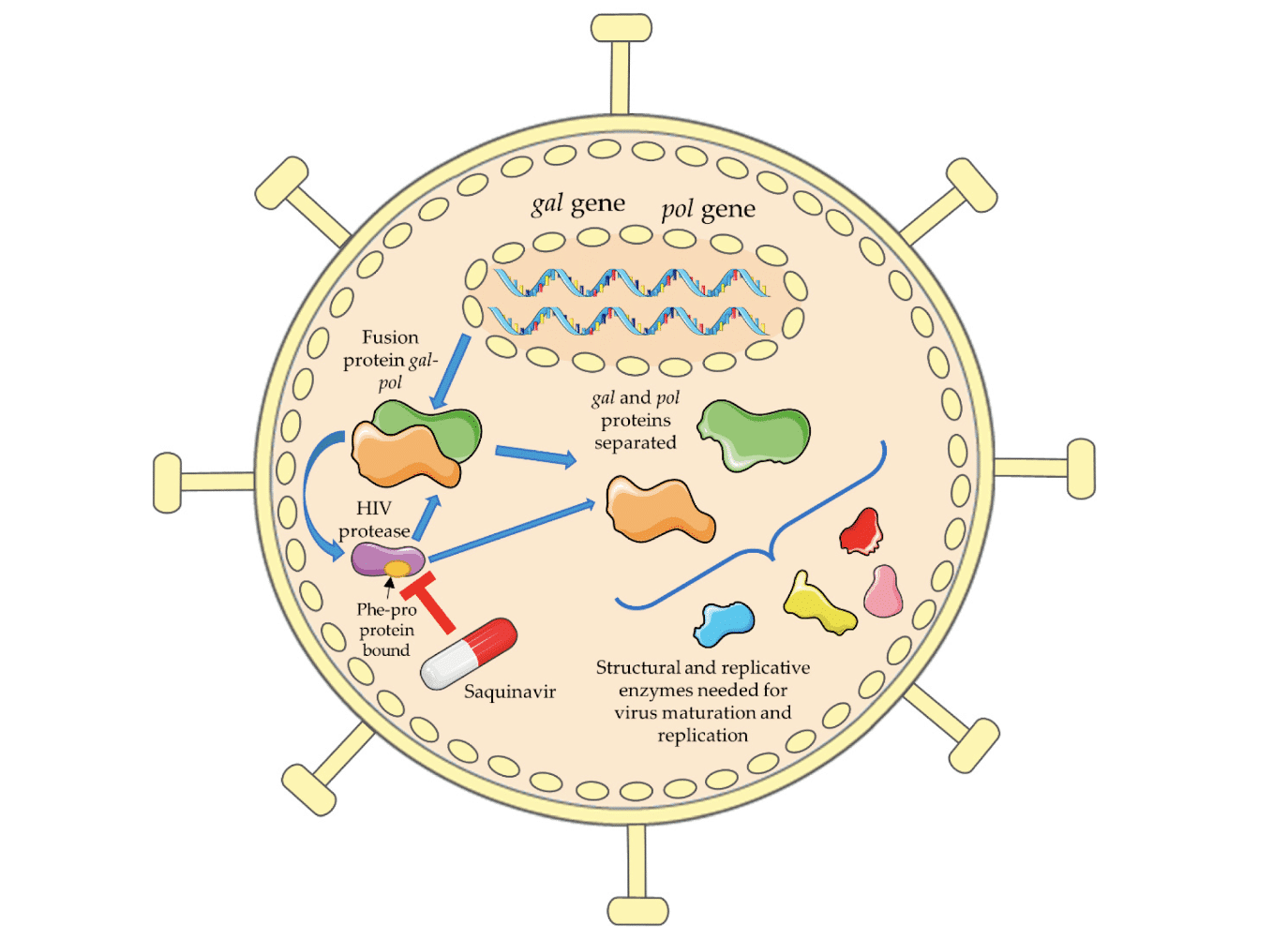
Figure 3: Protease inhibitors (PIs) like Saquinavir block the HIV protease from creating functional proteins out of fused polyproteins. Photo credit to Mariana Pereira and Nuno Vale.
Protease inhibitors (PIs) could be co-administered alongside the NRTIs, blocking the HIV life cycle in two places at once, enhancing viral suppression, and making it harder for HIV to “mutate around” the combination of antiviral treatments.
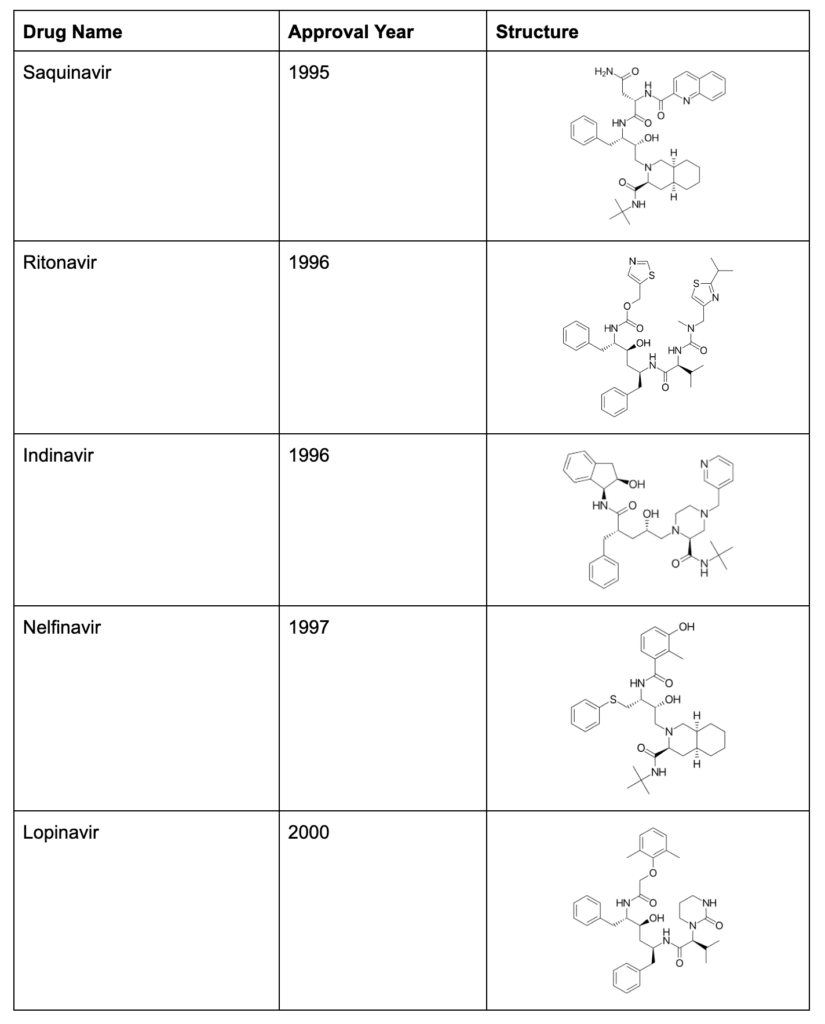
Non-Nucleoside Reverse Transcriptase Inhibitors
The normal flow of genetic information is DNA -> RNA -> Protein, but the reverse transcriptase (RT) enzyme reverses the flow of genetic information and gives HIV the ability to turn its RNA into a long-lasting DNA copy that the cell can read for its entire lifespan. RT is one of HIV’s most powerful tools, and there are two entire classes of medication that target it. NTRIs (like AZT, discussed above) target RT by tricking it into adding the NRTI to a stretch of HIV’s DNA. In 1996, Viramune was introduced as a new, direct way to inhibit RT by sticking to the enzyme directly, making it unable to turn HIV RNA into DNA. Viramune became the first of a new class of medications called Non-Nucleoside Reverse Transcriptase Inhibitors (NNRTIs).
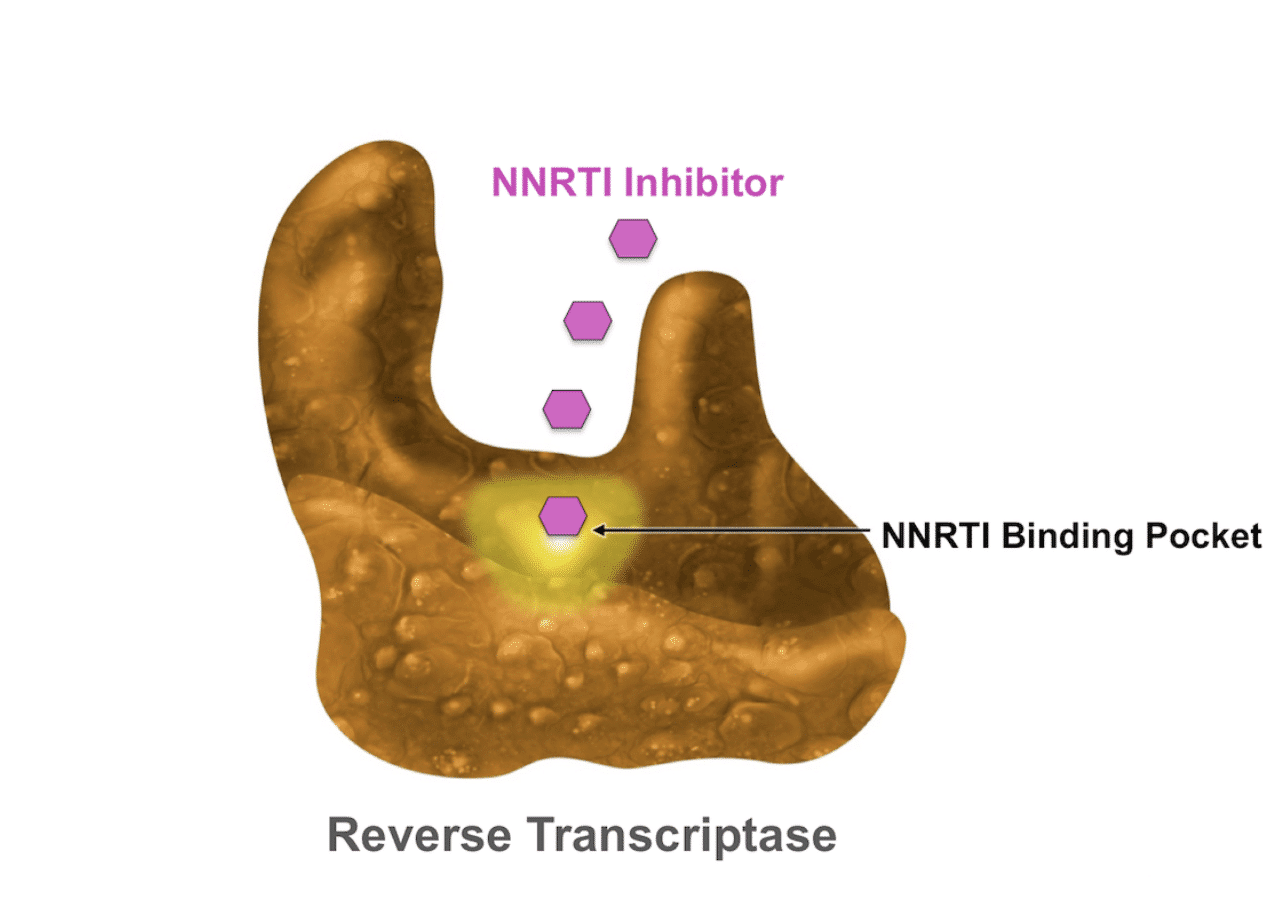
Figure 4: NNRTIs do not mimic nucleotides, instead they stick to the RT molecule and block it from working as intended. Photo credit to Brian Wood’s Evaluation and Management of Virologic Failure.
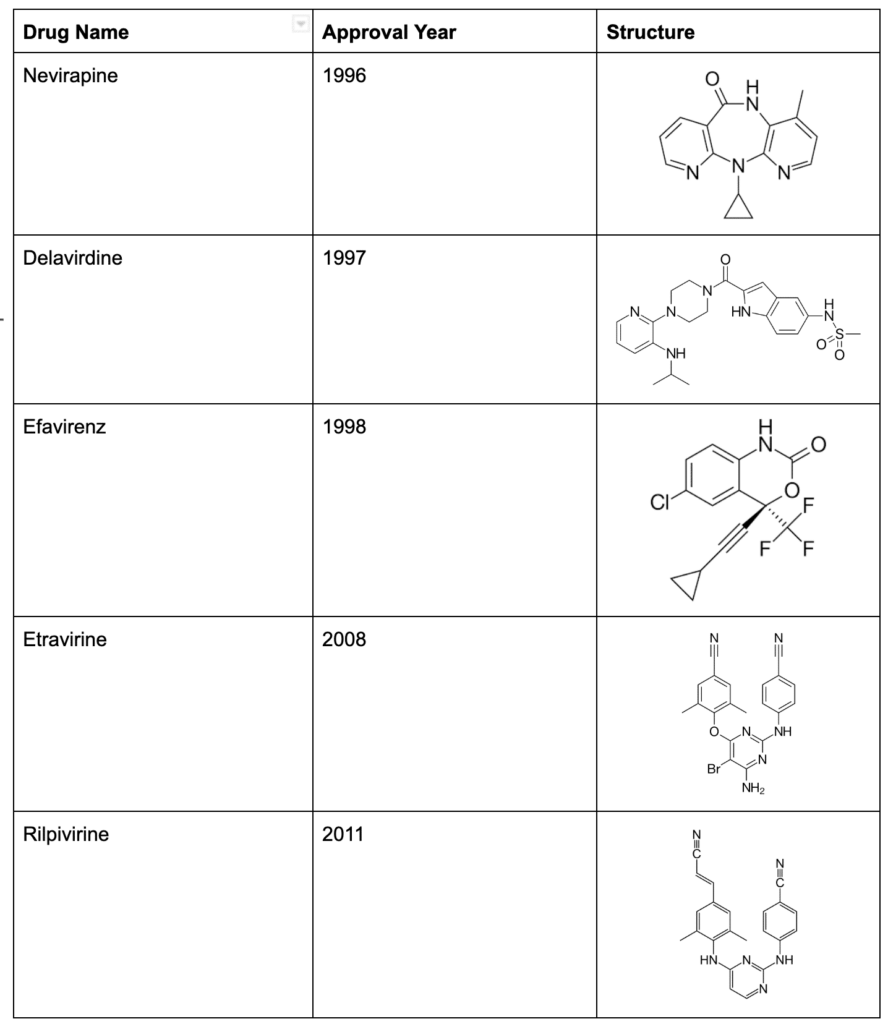
HAART
After the introduction of Viramune, pharmaceutical companies spent the next seven years continuously coming out with new NRTIs, NNRTIs, and PIs that improved upon the efficacy of their predecessors with better side-effect profiles. Highly Active Antiretroviral Therapy (HAART), known commonly as “the cocktail,” is a combination of multiple antiviral therapies, and became the standard of care in 1996. Mixing and matching the various antiviral drugs to create a personalized medication plan which could successfully suppress HIV while minimizing side effects became a specialized field in medicine.
Entry inhibitors – fusion inhibitors and coreceptor antagonists
In 2003, yet another method to break the viral cycle emerged. Instead of breaking the HIV life cycle during the assembly of HIV viral particles, Enfuviritide blocked HIV from fusing through the cell membrane, keeping it out of cells to prevent new cells from becoming infected in the first place. While the side effects of the medication and the need to inject it twice daily made its administration difficult, this drug was the first approved medication that kept HIV out of vulnerable cells, setting a trend which would be continued with the next class of antivirals.
Maraviroc was approved in 2007, giving doctors the ability to “cover” the receptors that HIV uses to grab onto the cell surface, preventing HIV from even attaching to cells. This strategy was refined with further additions to the entry receptor antagonists, expanding the methods that doctors could use to block HIV from infecting new cells in the body.
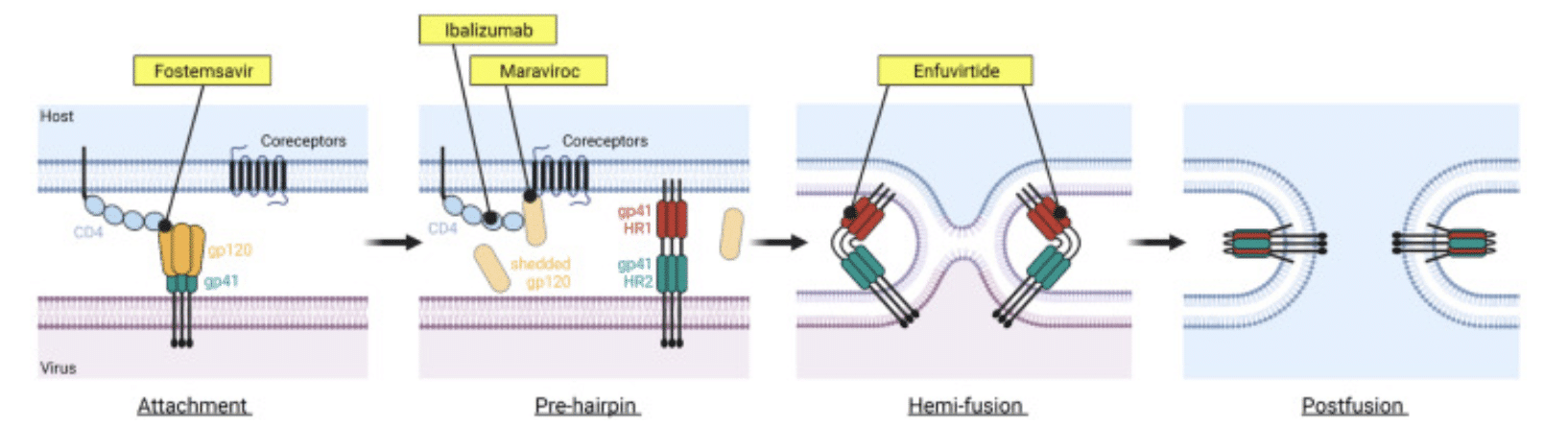
Figure 5: The stages of HIV entry are shown above, alongside points where antivirals inhibit viral entry.
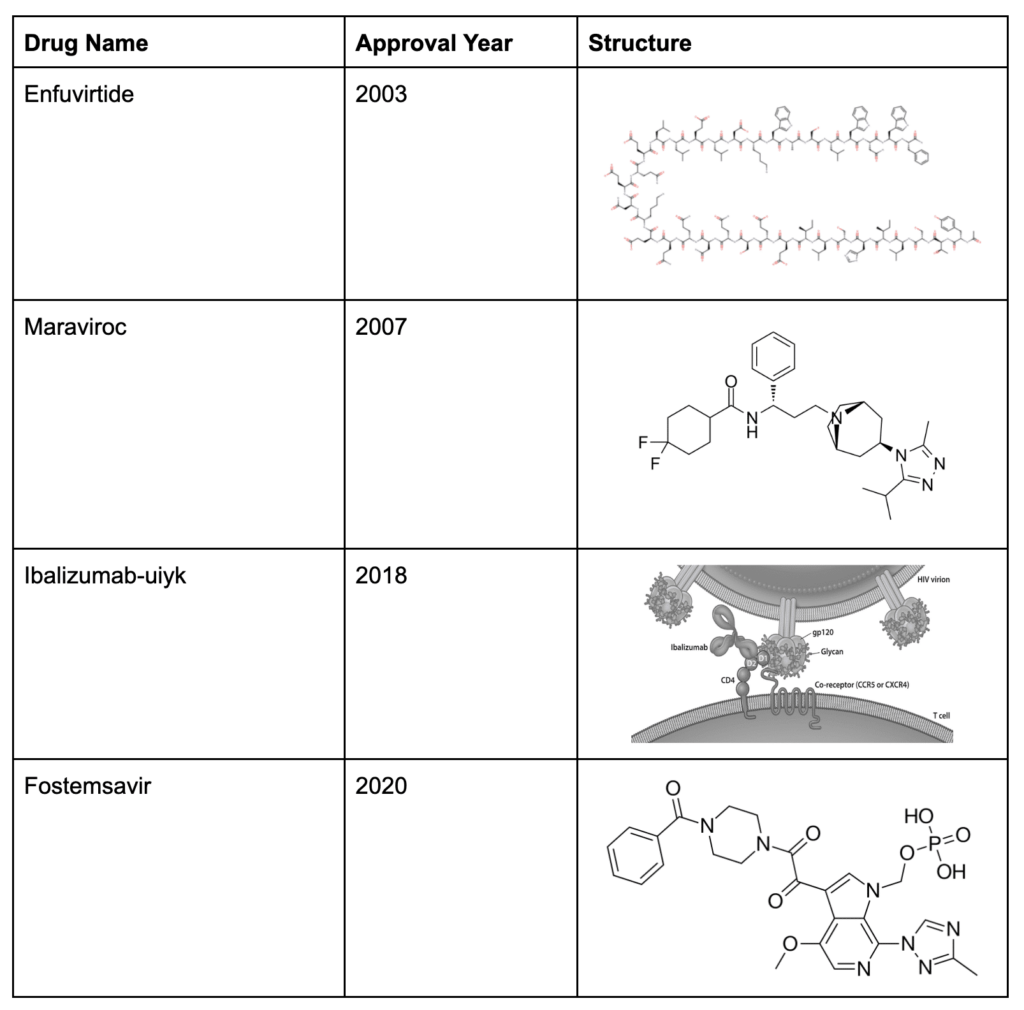
Integrase inhibitors
The final class of anti-HIV medication are the integrase inhibitors, which was founded in 2007 by Raltegravir. Similar to NRTIs and NNRTIs, the integrase inhibitors target a powerful enzyme that HIV uses to manipulate its genetic material. Integrase, as the name implies, integrates the HIV genome into the host cell’s genome, pictured below.
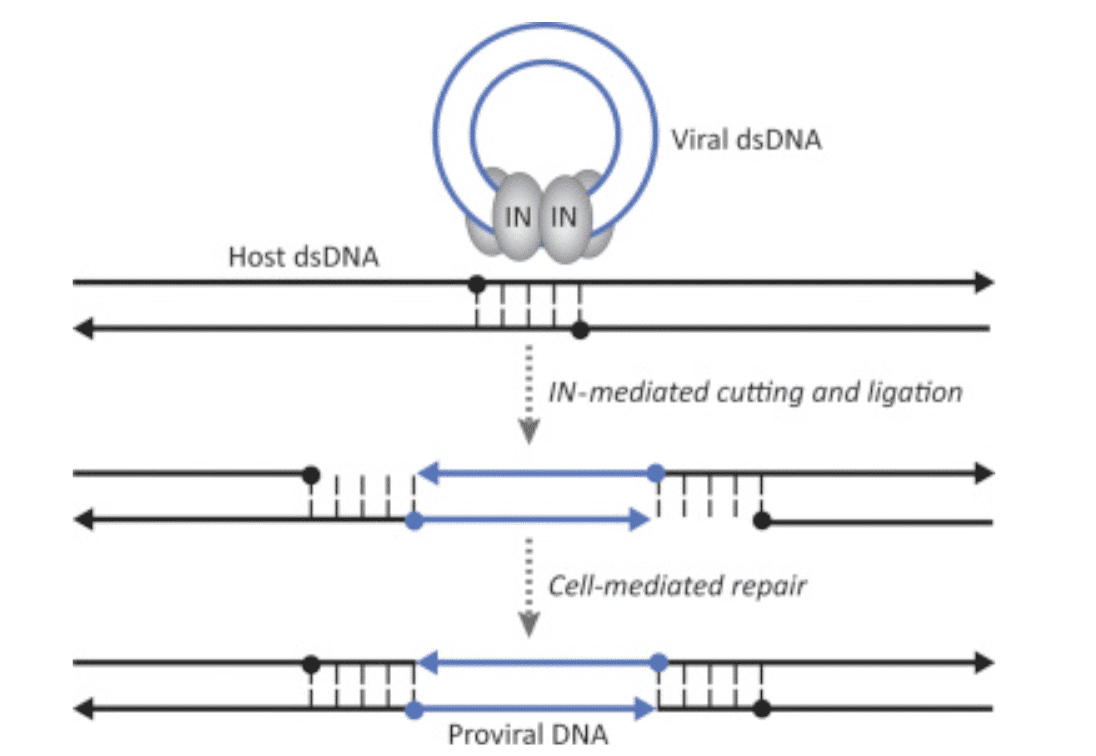
Figure 6: When RT has built a double stranded DNA copy of the HIV genome, integrase (labeled as “IN”) places the viral DNA inside the host cell’s DNA, making it difficult for the cell to discern what is “self DNA” and what is “viral DNA”.
Integration of the viral genome is required to complete the viral life cycle, so inhibiting integrase represents a potent antiviral approach. Inhibiting HIV at this step also helps prevent HIV from establishing new cells in the viral reservoir – since cells with integrated HIV genomes copy the HIV genome when they divide, their daughter cells are automatically infected with HIV. By blocking HIV from interweaving its genome with the host cell’s DNA, it is possible to inhibit this vicious cycle.
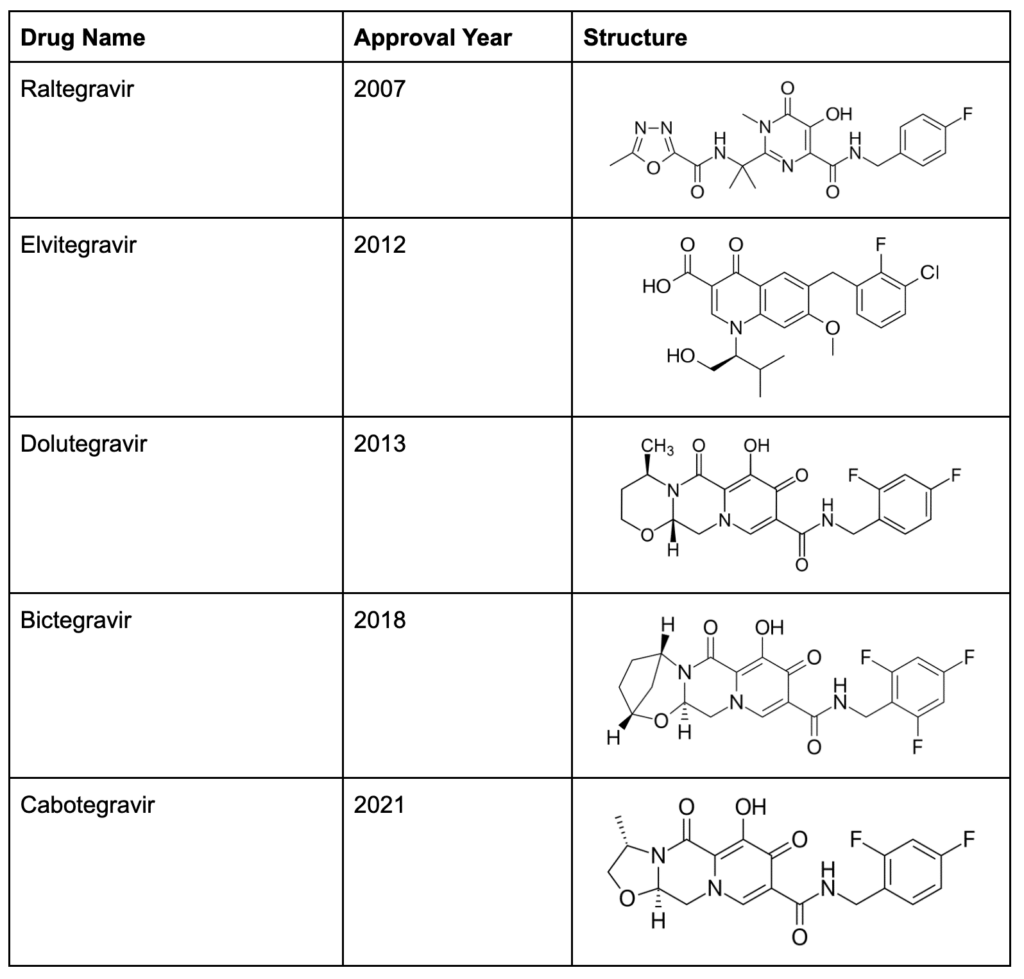
Gene therapy for HIV
Antivirals have proven that blocking the HIV life cycle in various places can bring viral replication to a halt, or at least block it so effectively that even advanced biochemical assays cannot detect viral RNA. These medications are highly effective, especially in combination, but they need to be taken for life, and they do still have side effects. The next logical step is to create a permanent solution to HIV infection, which is where gene therapy comes in. Gene therapy has already been used successfully for cancer and inherited diseases. In HIV, Sangamo’s studies on SB-728-T used early gene editing techniques in an attempt to create a functional cure for HIV. While they saw limited success, with one trial participant who has remained off of ART since 2014, development on SB-728-T was discontinued due to the low percentage of participants who showed durable viral suppression.
Addimmune believes that gene therapy is an iterative technology – that continually learning from past experiments and improving upon their design will yield a cure. We are currently in human trials for AGT103-T, a gene therapy for HIV, which teaches cells how to break the HIV life cycle without the help of antiviral medication. With the help of AGT103, T cells that would normally be susceptible to HIV are made resistant to infection, thus giving the immune system a chance to fight HIV like any other pathogen. Here’s how it works:
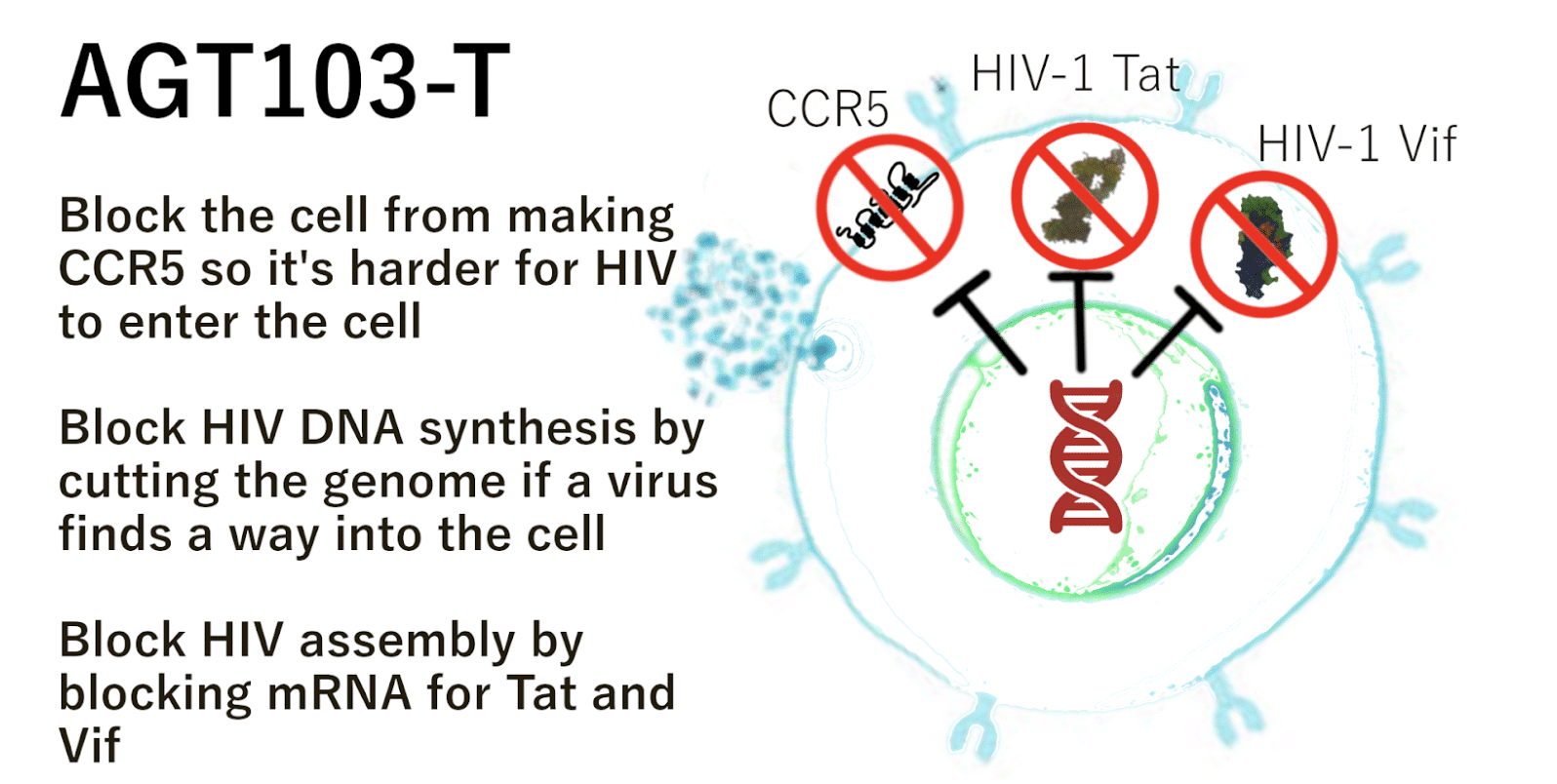
Figure 7: AGT103-T is made of modified T cells that can block the HIV life cycle in multiple ways, similar to a HAART regimen. Instead of taking multiple chemical antivirals, these cells have a specialized anti-HIV gene. This allows them to do their job as immune cells without being killed by HIV.
AGT103-T was designed to induce the immunologic conditions seen in the world’s few HIV cure cases. By placing anti-HIV immune cells in the body and giving them a set of genetic instructions that can be used to block HIV replication, we can “install” HIV resistant cells which can coordinate an immune response against HIV without becoming infected themselves. These modified T cells tip the scales in favor of the immune system and open the possibility of hunting down HIV infected cells instead of simply blocking infected cells from producing viable copies of HIV.